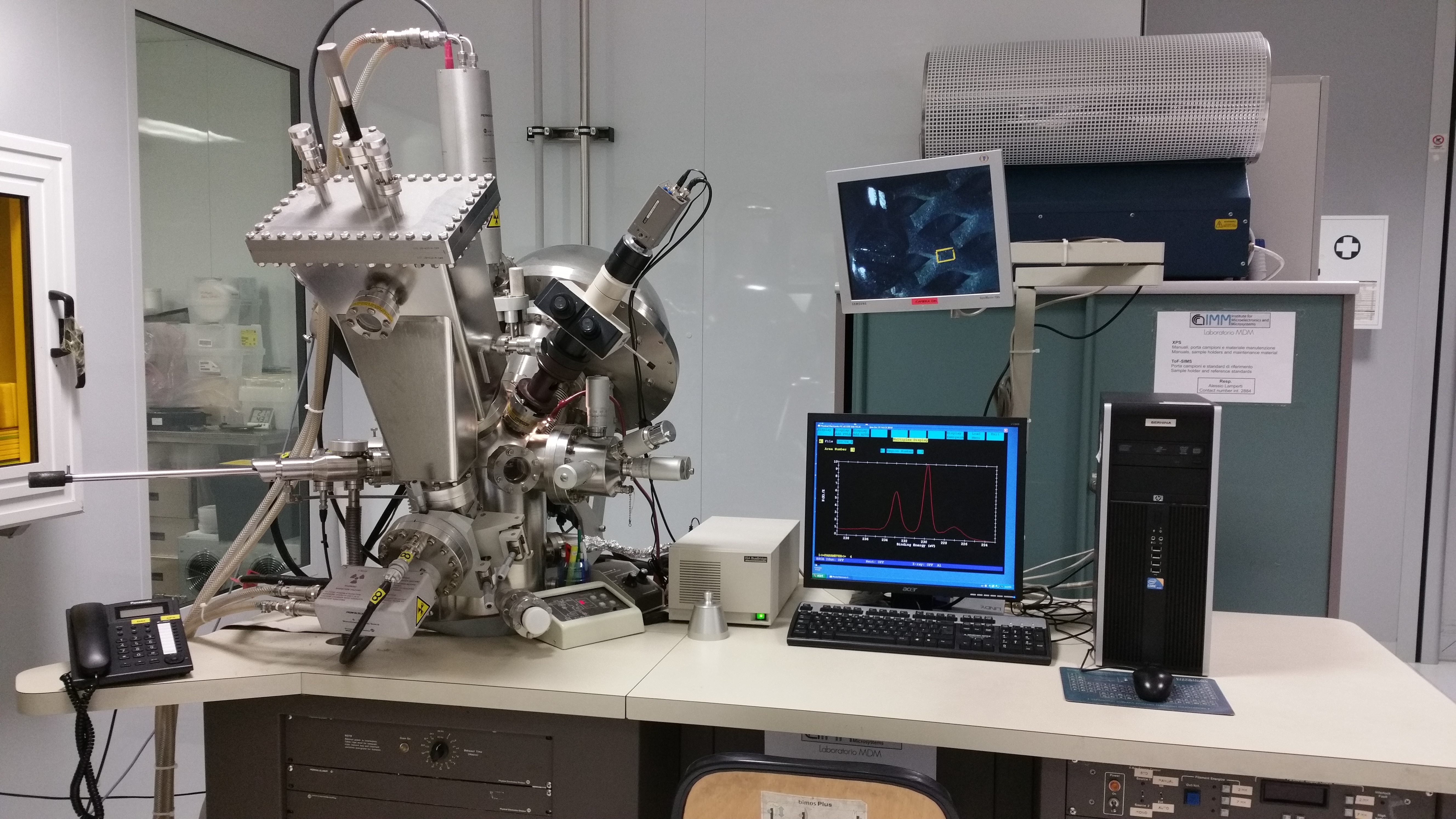
Spettroscopia di fotoelettroni da raggi X (XPS)
XPS è una tecnica di spettroscopia fotoelettronica utilizzata per sondare le superfici dei materiali. Essa consente di conoscere gli elementi chimici che compongono la superficie di un materiale e di determinarne lo stato di legame.
Descrizione
Principio di funzionamento
Il campione viene irraggiato con una sorgente di raggi X monocromatica. I fotoni entrano nel materiale e subiscono varie interazioni, tra le quali l’effetto fotoelettrico e l’emissione Auger. In entrambi i casi un elettrone viene espulso dal materiale con una energia cinetica legata alla energia di legame dello stesso.
Misurando l’energia cinetica dell’elettrone espulso si risale alla sua energia di legame, indicativa dell’elemento chimico interessato, secondo la formula:
dove è l’energia di legame,
l’energia dei fotoni incidenti,
l’energia cinetica dell’elettrone e
la funzione lavoro dello spettrometro.
Nel caso di analisi di un solido, la tecnica permette di analizzarne i primi strati atomici (una profondità di qualche nanometro), poiché solo gli elettroni eccitati in prossimità della superficie riescono a fuoriuscire dal campione senza subire interazioni con conseguenti perdite di energia e quindi mantenendo l’informazione che trasportano.
Strumento
Sorgente a raggi X
Come sorgente viene utilizzato un tubo a raggi X. Esso è composto da un filamento attraversato da corrente e tenuto a un potenziale più negativo rispetto a una griglia che gli sta davanti. Il filamento emette elettroni per effetto termoionico, questi vengono accelerati verso l’anodo per la differenza di potenziale e vanno a collidere contro una targhetta di metallo (generalmente alluminio) posta in prossimità della griglia (l’anodo). Gli elettroni penetrano all’interno della targhetta e perdono energia per vari fenomeni. Tra i vari vi è la perdita di energia per frenamento (interazione degli elettroni con i nuclei atomici) che causa emissione di raggi X (ogni particella carica sottoposta ad accelerazione emette radiazione elettromagnetica), e la emissione di raggi X caratteristici. Questa avviene quando l’elettrone subisce un urto anelastico e provoca lo scalzamento di un elettrone della targhetta che lascia una vacanza alle sue spalle.
In un tempo breve (il tempo di rilassamento dell’atomo) un altro elettrone va ad occupare la vacanza da un livello più esterno o dal livello di vuoto attraverso una transizione radiativa cui è associata l’emissione di un fotone di energia pari alla differenza di energia dei due livelli atomici coinvolti.
Analizzatore
Uno degli analizzatori più usati è il settore elettrostatico. Un altro è il campo ritardante.
Applicazioni
La tecnica trova un utilizzo abbastanza ampio in campo industriale, è particolarmente utile nel campo della catalisi dal momento che è fondamentale conoscere lo stato della superficie dei catalizzatori.
Essendo una tecnica non distruttiva trova anche un buon utilizzo nel campo dei beni culturali.
Voci correlate
Campo di applicazione
Applicazioni
La tecnica trova un utilizzo abbastanza ampio in campo industriale, è particolarmente utile nel campo della catalisi dal momento che è fondamentale conoscere lo stato della superficie dei catalizzatori.
Essendo una tecnica non distruttiva trova anche un buon utilizzo nel campo dei beni culturali.
Prestazioni
Routine limits
Quantitative accuracy and precision
- XPS is widely used to generate an empirical formula because it readily yields excellent quantitative accuracy from homogeneous solid-state materials.
- Quantification can be divided into two categories: absolute quantification and relative quantification. The former generally requires the use of certified (or independently verified) standard samples, is generally more challenging, and is generally less common.
- Relative quantification is more common and involves comparisons between several samples in a set for which one or more analytes are varied while all other components (the sample matrix) are held constant.
- Quantitative accuracy depends on several parameters such as: signal-to-noise ratio, peak intensity, accuracy of relative sensitivity factors, correction for electron transmission function, surface volume homogeneity, correction for energy dependence of electron mean free path, and degree of sample degradation due to analysis.
- Under optimum conditions, the quantitative accuracy of the atomic percent (at%) values calculated from the Major XPS Peaks is 90-95% for each major peak. If a high level quality control protocol is used, the accuracy can be further improved.
- Under routine work conditions, where the surface is a mixture of contamination and expected material, the accuracy ranges from 80-90% of the value reported in atomic percent values.
- The quantitative accuracy for the weaker XPS signals, that have peak intensities 10-20% of the strongest signal, are 60-80% of the true value, and depend upon the amount of effort used to improve the signal-to-noise ratio (for example by signal averaging).
- Quantitative precision (the ability to repeat a measurement and obtain the same result) is an essential consideration for proper reporting of quantitative results. Standard statistical tests, such as the Student’s t test for comparison of means, should be used to determine confidence levels in the average value from a set of replicate measurements, and when comparing the average values of two or more different sets of results. In general, a p value (an output of the Student’s t test) of 0.05 or less indicates a level of confidence (95%) that is accepted in the field as significant.
Analysis time
- Typically ranging 1–20 minutes for a broad survey scan that measures the amount of all detectable elements, typically 1–15 minutes for high resolution scan that reveal chemical state differences (for a high signal/noise ratio for count area result often requires multiple sweeps of the region of interest), 1–4 hours for a depth profile that measures 4–5 elements as a function of etched depth (this process time can vary the most as many factors will play a role).
Detection limits
Detection limits may vary greatly with the cross section of the photoelectron line of interest and the background signal level which is a function of the matrix material. In general photoelectron cross sections increase with atomic number, while the background is a function of the composition of the matrix material and the binding energy. Background signals generally increase with atomic number of the matrix material and decrease with increasing kinetic energy. For example in the case of gold on silicon where the high cross section Au4f peak is at a higher kinetic energy than the major silicon peaks, it sits on a very low background and detection limits of 1ppm or better may be achieved with reasonable acquisition times. Conversely for silicon on gold, where the modest cross section Si2p line sits on the large background below the Au4f lines, detection limits would be much worse for the same acquisition time. Detection limits are often quoted as 0.1–1.0 % atomic percent (0.1 % = 1 part per thousand = 1000 ppm) for practical analyses, but lower limits may be achieved in many circumstances.
Measured area
- Measured area depends on instrument design. The minimum analysis area ranges from 10 to 200 micrometres. Largest size for a monochromatic beam of X-rays is 1–5 mm. Non-monochromatic beams are 10–50 mm in diameter. Spectroscopic image resolution levels of 200 nm or below has been achieved on latest imaging XPS instruments using synchrotron radiation as X-ray source.
Sample size limits
Instruments accept small (mm range) and large samples (cm range), e.g. wafers. Limiting factor is the design of the sample holder, the sample transfer, and the size of the vacuum chamber. Large samples are laterally moved in x and y direction to analyse a larger area.[citation needed]
Degradation during analysis
- Depends on the sensitivity of the material to the wavelength of X-rays used, the total dose of the X-rays, the temperature of the surface and the level of the vacuum. Metals, alloys, ceramics and most glasses are not measurably degraded by either non-monochromatic or monochromatic X-rays. Some, but not all, polymers, catalysts, certain highly oxygenated compounds, various inorganic compounds and fine organics are degraded by either monochromatic or non-monochromatic X-ray sources.
- Non-monochromatic X-ray sources produce a significant amount of high energy Bremsstrahlung X-rays (1–15 keV of energy) which directly degrade the surface chemistry of various materials. Non-monochromatic X-ray sources also produce a significant amount of heat (100 to 200 °C) on the surface of the sample because the anode that produces the X-rays is typically only 1 to 5 cm (2 in) away from the sample. This level of heat, when combined with the Bremsstrahlung X-rays, acts synergistically to increase the amount and rate of degradation for certain materials. Monochromatic X-ray sources, because they are far away (50–100 cm) from the sample, do not produce any heat effects.
- Monochromatic X-ray sources are monochromatic because the quartz monochromator system diffracts the Bremsstrahlung X-rays out of the X-ray beam, which means the sample is only exposed to one narrow band of X-ray energy. For example, if aluminum K-alpha X-rays are used, the intrinsic energy band has a FWHM of 0.43 eV, centered on 1,486.7 eV (E/ΔE = 3,457). If magnesium K-alpha X-rays are used, the intrinsic energy band has a FWHM of 0.36 eV, centered on 1,253.7 eV (E/ΔE = 3,483). These are the intrinsic X-ray line widths; the range of energies to which the sample is exposed depends on the quality and optimization of the X-ray monochromator.
- Because the vacuum removes various gases (e.g., O2, CO) and liquids (e.g., water, alcohol, solvents, etc.) that were initially trapped within or on the surface of the sample, the chemistry and morphology of the surface will continue to change until the surface achieves a steady state. This type of degradation is sometimes difficult to detect.
General summary of use
- XPS is, in effect, a non-destructive technique that measures the surface chemistry of most any material, however non-dry, outgassing, radioactive or highly magnetic materials can pose serious challenges.
Bibliografia
Practical Surface Analysis, Auger and X-ray Photoelectron Spectroscopy